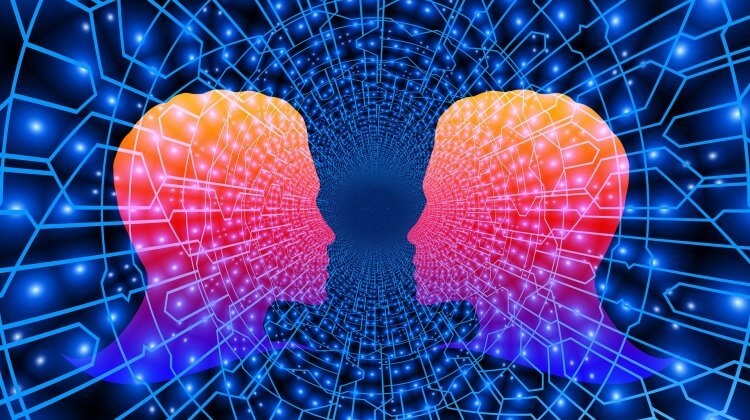
Estimated reading time: 17 minutes
Table of contents
- Introduction
- The Hypothalamus is the Master Regulator of the HPGA
- Negative feedback of the HPGA by sex steroids
- Progesterone and its derivatives can also provide negative feedback to the HPGA
- Elevated prolactin often causes symptoms of hypogonadism, but its suppressive effect on testosterone is less obvious
- A (large) energy deficit can suppress testosterone levels and other stressors can compound this
- References
Introduction
Most people are aware that testosterone, in men, is produced by the testes. A lot of people are also aware that this is the result of stimulation of the Leydig cell in the testes by a hormone called luteinizing hormone (LH). In turn, this hormone is produced in response to another hormone called gonadotropin-releasing hormone (GnRH). Or summarized:
GnRH → LH → testosterone
Most anabolic steroid users are aware that anabolic steroids suppress the endogenous testosterone production. But how does this work? How is this regulated? That will be the topic of this article. Let’s take a deep dive into the hormonal axis that regulates testosterone production: the hypothalamic-pituitary-gonadal axis (HPGA).
The Hypothalamus is the Master Regulator of the HPGA
The hypothalamus is a small part of the brain. It’s located at the base of the brain, close to the pituitary that is also part of the HPGA. Despite its relatively small size, it’s crucial for life. Without a hypothalamus, you’re dead.
It integrates a lot of signals, both neural as well as endocrine in nature. Meaning that it receives signals from other parts of the brain through neurons, as well as through hormones that are produced more remotely in the body. It is also responsible for the regulation of several hormonal axes. In addition to the regulation of the HPGA, it also regulates the production of the following hormones as part of a hormonal axis:
- cortisol production as part of the hypothalamic-pituitary-adrenal axis (HPAA)
- thyroid hormones production as part of the hypothalamic-pituitary-thyroid axis (HPTA)
- growth hormone and insulin-like growth factor 1 production as part of the hypothalamic-pituitary-somatotropic axis (HPSA)
It, ultimately, regulates these various hormonal axes through the secretion of so-called releasing hormones. As part of the HPAA, the releasing hormone is corticotropin-releasing hormone (CRH). As part of the HPTA it’s thyrotropin-releasing hormone (TRH), and as part of the HPSA it’s growth hormone-releasing hormone (GHRH). And, as introduced earlier in this article, as part of the HPGA it’s GnRH. This hormone binds to its receptor, the GNRH receptor, on the gonadotropic cells of the anterior pituitary. When this happens, the gonadotropic cells will secrete gonadotropins: LH & FSH.
It’s important to consider how GnRH secretion is regulated. As this is, by far, the most complex part of the HPGA.
The secretion of GnRH takes place in distinct pulses. It’s not continuously secreted, it’s secreted in a pulsatile fashion. This pulsatility is important for its functioning for two main reasons. The first reason is that continuous secretion of GnRH would, with time, completely blunt its effect on the gonadotropic cells of the anterior pituitary. Point in case, if you administer a long-acting GnRH agonist daily, you’ll end up severely suppressing the release of LH and FSH—and consequently testosterone [1]. The other reason is that the pulse frequency of GnRH affects the ratio at which LH and FSH are secreted. A slower pulse frequency favors the secretion of FSH, whereas a faster one favors the secretion of LH [2]. This has been demonstrated most elegantly in an animal model (rhesus monkeys) [3]. (You can imagine the ethical objections to doing this in humans. But there’s a plethora of evidence indicating that a similar biological effect of GnRH pulsatility takes place in humans.)
For the purpose of demonstrating this, the researchers had inflicted a hypothalamic lesion in the monkeys so they could replace their endogenous GnRH production with a GnRH infusion. This infusion enabled the researchers to control its pulsatility perfectly. In the picture below you can see how a shift from 1 GnRH pulse an hour, to 1 pulse every 3 hours, dramatically decreased the LH levels and increased the FSH levels. When the pulsality was restored afterwards, the FSH and LH levels recovered again over the course of several days to their previous levels.
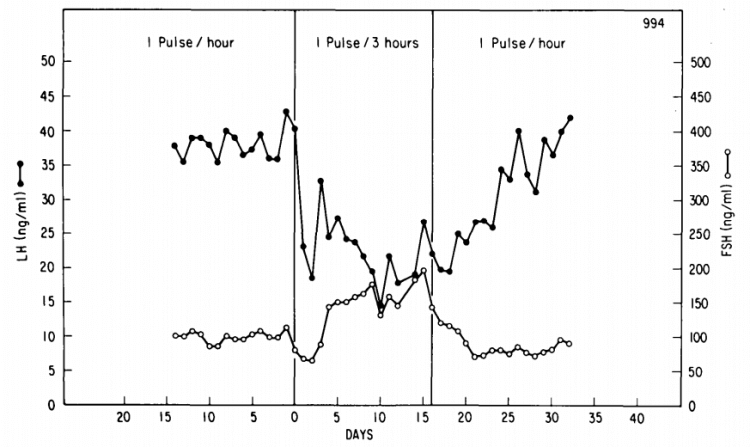
The GnRH pulsatility, in turn, is caused by another group of neurons. More specifically, by so-called KNDy neurons. They derive their name by three peptides that they secrete and a responsible for the pulsatility. The K stands for kisspeptin. The N stands for neurokinin B, and the Dy stands for dynorphin. The KNDy neurons possess intrinsic and synchronized pulsatility. How this is established is proposed in a model by Navarro et al. in 2009 [4].
For my first (Dutch) book about anabolic steroids, which I published in 2015, I drew the following picture to capture this model by Navarro et al.
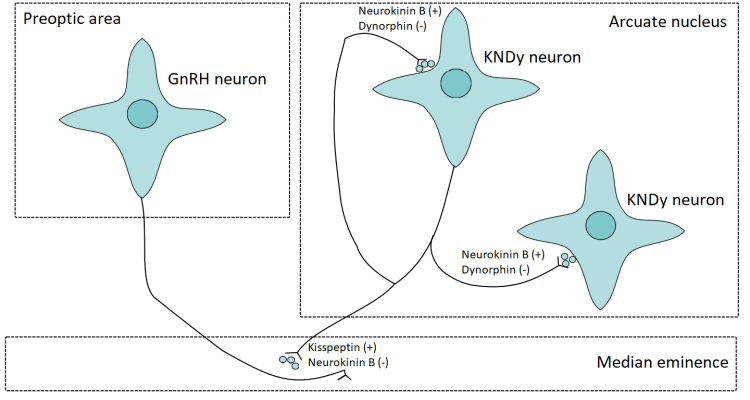
Let me explain what’s happening and what you’re looking at. The GnRH neurons, displayed on the left, are dispersed throughout an area of the hypothalamus called the preoptic area (POA). This is where their cell bodies reside: those ‘star-like’ looking figures. Their axons, the black lines, project into the median eminence. Axons are responsible for passing on the signal from a neuron to another cell. In this case, these axons are also called ‘dendrons’, as they are said to combine the function of axons (propagating a signal) as well as receiving input (as dendrites would do).
The median eminence is a part of the hypothalamus in which the releasing hormones mentioned earlier are released. It’s connected to the pituitary with the hypophyseal portal system. Thus the blood vessels there are able to carry these releasing hormones to the pituitary in order for them to exert their action.
On the right you see the KNDy neurons. These can be found in a part of the hypothalamus called the arcuate nucleus in rodents (in humans the equivalent would be the infundibular nucleus). When they become (spontaneously) active, they’ll start to secrete kisspeptin, neurokinin B and dynorphin. Neurokinin B amplifies this effect and also propagates the signal to other KNDy neurons that are in close proximity to enhance synchronicity of the cluster of KNDy neurons. The kisspeptin that is released into the median eminence acts on the GnRH dendrons to stimulate GnRH secretion. With some delay, the dynorphin is released. Dynorphin inhibits the KNDy neuron activity, thus terminating the effect. Additionally, neurokinin B also seems necessary to act on the GnRH dendrons. The reason for this is that kisspeptin has a prolonged effect. Thus it is postulated that neurokinin B action on the GnRH dendrons, which is also released in pulsatile fashion, is responsible for the subsequent pulsatile release of GnRH. And, indeed, when you administer kisspeptin to adult men, they show a robust increase in LH & FSH levels [5].
Negative feedback of the HPGA by sex steroids
A bunch of future studies have further largely confirmed and refined this model of pulsatile GnRH secretion [6]. Importantly, this model explains how negative feedback by, for example androgens and estrogens, affects GnRH secretion. After all, evidence seems to indicate that GnRH neurons lack both the androgen receptor (AR) and estrogen receptor (ER) [7]. In contrast, the KNDy neurons do express these receptors. Thus, this population of neurons could function as a relay through which androgens and estrogens provide negative feedback at the level of the hypothalamus.
Both androgens and estrogens slow down the rate of GnRH pulse frequency [8]. Interestingly, estrogens, but not androgens, provide negative feedback at the pituitary. As such, testosterone is dependent on its aromatization into estradiol for its complete inhibitive effect on LH secretion. Nevertheless, the dominant site of negative feedback seems to lie at the hypothalamus.
Further underscoring estradiol’s potency in inhibiting gonadotropin secretion is that it’s estimated to be about 200 times more effective at this than testosterone molecule per molecule [9]. However, this number should be placed into context. Of course, serum estradiol levels are a lot lower than that of testosterone, too. When looking at the free fraction of both testosterone and estradiol, one study found serum concentrations of 138.8 and 0.5 pg/mL, respectively, in young men [10]. Or in other words, the serum free testosterone concentration was roughly 200-300 times higher than that of free estradiol too. While aromatase is expressed in the pituitary, evidence indicates that aromatization in situ at the pituitary is not necessary and possibly not even required, as circulating estrogen seems to be the main driver [8].
And, indeed, when the conversion of testosterone to estradiol is blocked by an aromatase inhibitor, a strong increase in LH and testosterone can be observed [11]. Similarly, selective estrogen receptor modulators (SERMs) which function as an ER antagonist at the level of the hypothalamus/pituitary, such as clomiphene, also strongly increase LH and testosterone [12].
When looking at the nonaromatizing androgen stanozolol, it’s clear how ‘pure’ androgens can suppress testosterone production too. Subjects receiving the compound for 2 weeks saw their free testosterone drop by 43% and their LH by 31% [13]. A relatively high dosage (80 mg daily) of oxandrolone (Anavar, also a nonaromatizing androgen), led to a modest 31% decrease in free testosterone after 12 weeks in HIV-infected men [14]. A trial in 79 HIV-infected men randomized to 50 mg oxymetholone 2x daily, 50 mg oxymetholone 3x daily, or a placebo, noted marked suppression of testosterone and LH [15]. Total testosterone decreased by 68% in the 150 mg group and 71% in the 100 mg group. LH decreased by 52% and 65%, respectively. The absence of full suppression, as well as the apparent lack of stronger suppression in the higher dosage group, seem to suggest that sole androgen suppression might be unable to lead to complete suppression.
Progesterone and its derivatives can also provide negative feedback to the HPGA
In addition to sex steroids, progesterone is also capable of suppressing gonadotropin secretion. Because of this, the addition of a progesterone derivative to testosterone therapy has been explored in the area of male contraception. In one study, healthy men were randomized to receive either testosterone enanthate (100 mg weekly), or the same dosage of testosterone in combination with the progestogen levonorgestrel [16]. While there clearly was some residual LH and FSH secretion in the men receiving testosterone only, the addition of levonorgestrel virtually abolished this residual secretion.
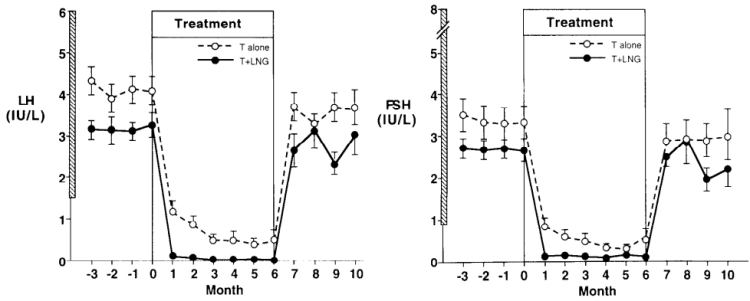
In a similar vein, one might expect a stronger suppressive effect of anabolic steroids that are capable of binding, and activating, the progesterone receptor. Some examples of this would be mibolerone, methyltrienolone, trenbolone and nandrolone, as they demonstrate agonistic activity for the progesterone receptor in a mammalian reporter gene bioassay [17]. However, it remains hard to extrapolate this to in vivo suppression in humans. For example, nandrolone hexyloxyphenylpropionate (which has a very long half-life of about 21 days [18]) at a dosage of 200 mg every 3 weeks—and a 400 mg loading dose—failed to maintain full gonadotropin suppression that was induced by a 12-week combined administration period of a GnRH antagonist and nandrolone [19].
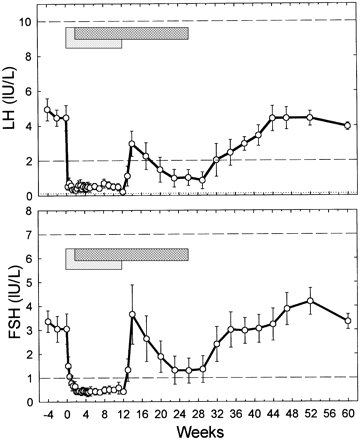
GnRH neurons also lack progesterone receptors, just they lack sex steroids receptors. However, KNDy neurons are found to express progesterone receptors in various species [20]. As such, similar to sex steroids, it’s assumed negative feedback is mediated by the KNDy neurons. While human data is lacking, some data from animal experiments suggests that suppression by progesterone also takes place at the pituitary [21].
Elevated prolactin often causes symptoms of hypogonadism, but its suppressive effect on testosterone is less obvious
Another hormone that can suppress testosterone production is prolactin.In women, prolactin production is stimulated in response to breastfeeding. Or I should say, suckling on the nipple. Prolactin, in turn, works sort of as a natural contraceptive while breastfeeding, as it disrupts GnRH release (lactational amenorrhea) [22]. Based on animal data, it is assumed the effect is mediated via the KNDy neurons rather than the GnRH neurons [23]. And while prolactin can act on the testes and pituitary, it’s assumed that it’s main effect indeed is at the level of the hypothalamus [24]. This mostly stems from data which demonstrates that pulsatile GnRH replacement restores functioning of the HPG axis or fertility.
So how suppressive is it for testosterone production? Most data available stems from men with overt hyperprolactinemia. These men often, but definitely not always, show low testosterone levels. This picture is confounded by the finding that men with hyperprolactinemia often appear to have suppressed SHBG and subsequently normal free testosterone levels [25]. However, symptoms of hypogonadism are commonly present—even in the absence of any testosterone deficiency. Nevertheless, it’s fair to say that mild elevations usually don’t significantly impact testosterone levels.
While no value should be attributed to it, I’d like to fit in an anecdote of mine. One of my clients had a prolactin value around 450 ng/mL (lab reference range 3.46 – 19.40 ng/mL), which turned out to be caused by a prolactinoma. Despite this strongly increased prolactin value, he had a normal testosterone value of 501 ng/dL (17 nmol/L), and free testosterone value of 11.4 ng/dL. As expected, his gonadotropins didn’t show any abnormal levels either (FSH: 2 IU/L and LH 3.2 IU/L).
A (large) energy deficit can suppress testosterone levels and other stressors can compound this
As well known, certain stressors can also impact testosterone levels. One of them being an energy deficit. While a short-term (4-day) large energy deficit (62.5%) didn’t significantly decrease serum testosterone in a small group of men [26], a 48-hour fast led to a 21% reduction [27], and a 83-hour fast led to a 30% reduction [28]. Since the number of LH pulses decreased under these conditions, it’s very likely this is a consequence of a slowing of the GnRH pulse generator. As such, a (severe) decrease in energy availability seems to act on the hypothalamus.
In another trial, 4 days of (heavy) military exercise combined with a large energy deficit (60%) and only 4 hours of sleep per night led to a 49% reduction in serum testosterone [29]. Prolonging such conditions has the potential to decrease testosterone even more. A trial which followed subjects following an 8-week US Army Ranger training saw a decrease of 83% [30]. Testosterone went from 17.3 nmol/L to 3 nmol/L, that’s getting close to castration levels. In this trial, the subjects had an average energy deficit of 1,000 kcal daily, with peaks of 4,000 kcal. Combined with the 3.6 hours of sleep per night and the intense military training, this turned out to be a recipe for a solid drop in testosterone levels.
Highlighting the pivotal role of energy intake in this is an earlier US Army Ranger Training study [31]. Subjects were given a refeed during the training period, in which the other stressors were still present (military training and sleep deprivation). Testosterone made a stellar recovery during the refeed.
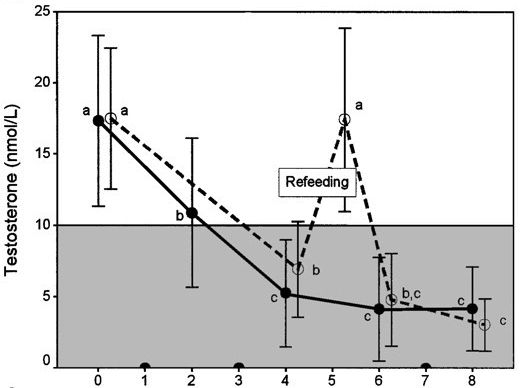
These results suggest that insufficient energy intake might be required for the other stressors (intense exercise and sleep deprivation) to affect testosterone levels.
Other stressors might affect testosterone levels through activation of the hypothalamic-pituitary-adrenal axis (HPAA). The end product of this hormonal axis is the glucocorticoid hormone cortisol. Various experimental animal models, in which the HPAA was activated, demonstrate decreases in circulating levels of gonadotropins [32]. One can imagine the ethical objections with the execution of some of these experimental models in humans. However, one might have a peek at the, somewhat extreme, example of Cushing’s syndrome. Herein, cortisol levels are too high and low testosterone can be observed. The low testosterone resolves once cortisol levels are normalized again [33]. Similarly, chronic glucocorticoid therapy, which is required as a treatment for certain diseases, reduces testosterone levels too [34].
References
- Conn, P. Michael, and William F. Crowley Jr. “Gonadotropin-releasing hormone and its analogues.” New England Journal of Medicine 324.2 (1991): 93-103.
- Ferris, Heather A., and Margaret A. Shupnik. “Mechanisms for pulsatile regulation of the gonadotropin subunit genes by GNRH1.” Biology of reproduction 74.6 (2006): 993-998.
- Wildt, L., et al. “Frequency and amplitude of gonadotropin-releasing hormone stimulation and gonadotropin secretion in the rhesus monkey.” Endocrinology 109.2 (1981): 376-385.
- Navarro, Victor M., et al. “Regulation of gonadotropin-releasing hormone secretion by kisspeptin/dynorphin/neurokinin B neurons in the arcuate nucleus of the mouse.” Journal of Neuroscience 29.38 (2009): 11859-11866.
- Dhillo, Waljit S., et al. “Kisspeptin-54 stimulates the hypothalamic-pituitary gonadal axis in human males.” The Journal of Clinical Endocrinology & Metabolism 90.12 (2005): 6609-6615.
- Herbison, Allan E. “The gonadotropin-releasing hormone pulse generator.” Endocrinology 159.11 (2018): 3723-3736.
- Oakley, Amy E., Donald K. Clifton, and Robert A. Steiner. “Kisspeptin signaling in the brain.” Endocrine reviews 30.6 (2009): 713-743.
- Pitteloud, Nelly, et al. “Inhibition of luteinizing hormone secretion by testosterone in men requires aromatization for its pituitary but not its hypothalamic effects: evidence from the tandem study of normal and gonadotropin-releasing hormone-deficient men.” The Journal of Clinical Endocrinology & Metabolism 93.3 (2008): 784-791.
- Hayes, Frances J., et al. “Aromatase inhibition in the human male reveals a hypothalamic site of estrogen feedback.” The Journal of Clinical Endocrinology & Metabolism 85.9 (2000): 3027-3035.
- Lakshman, Kishore M., et al. “The effects of injected testosterone dose and age on the conversion of testosterone to estradiol and dihydrotestosterone in young and older men.” The Journal of Clinical Endocrinology & Metabolism 95.8 (2010): 3955-3964.
- De Ronde, Willem, and Frank H. de Jong. “Aromatase inhibitors in men: effects and therapeutic options.” Reproductive Biology and Endocrinology 9.1 (2011): 1-7.
- Wheeler, Karen M., et al. “Clomiphene citrate for the treatment of hypogonadism.” Sexual medicine reviews 7.2 (2019): 272-276.
- Small, M., et al. “Alteration of hormone levels in normal males given the anabolic steroid stanozolol.” Clinical endocrinology 21.1 (1984): 49-55.
- Grunfeld, Carl, et al. “Oxandrolone in the treatment of HIV-associated weight loss in men: a randomized, double-blind, placebo-controlled study.” JAIDS Journal of Acquired Immune Deficiency Syndromes 41.3 (2006): 304-314.
- Hengge, Ulrich R., et al. “Double-blind, randomized, placebo-controlled phase III trial of oxymetholone for the treatment of HIV wasting.” Aids 17.5 (2003): 699-710.
- Bebb, Richard A., et al. “Combined administration of levonorgestrel and testosterone induces more rapid and effective suppression of spermatogenesis than testosterone alone: a promising male contraceptive approach.” The Journal of Clinical Endocrinology & Metabolism 81.2 (1996): 757-762.
- Houtman, Corine J., et al. “Detection of anabolic androgenic steroid abuse in doping control using mammalian reporter gene bioassays.” Analytica chimica acta 637.1-2 (2009): 247-258.
- Belkien, Lutz, et al. “Pharmacokinetics of 19-nortestosterone esters in normal men.” Journal of steroid biochemistry 22.5 (1985): 623-629.
- Behre, H. M., et al. “Suppression of spermatogenesis to azoospermia by combined administration of GnRH antagonist and 19-nortestosterone cannot be maintained by this non-aromatizable androgen alone.” Human Reproduction 16.12 (2001): 2570-2577.
- Moore, Aleisha M., et al. “KNDy cells revisited.” Endocrinology 159.9 (2018): 3219-3234.
- Girmus, Ronald L., and Mark E. Wise. “Progesterone directly inhibits pituitary luteinizing hormone secretion in an estradiol-dependent manner.” Biology of reproduction 46.4 (1992): 710-714.
- Tay, C. C. K., A. F. Glasier, and A. S. McNeilly. “The 24 h pattern of pulsatile luteinizing hormone, follicle stimulating hormone and prolactin release during the first 8 weeks of lactational amenorrhoea in breastfeeding women.” Human Reproduction 7.7 (1992): 951-958.
- Kokay, Ilona C., Sandra L. Petersen, and David R. Grattan. “Identification of prolactin-sensitive GABA and kisspeptin neurons in regions of the rat hypothalamus involved in the control of fertility.” Endocrinology 152.2 (2011): 526-535.
- Grattan, David R. “60 years of neuroendocrinology: the hypothalamo-prolactin axis.” Journal of Endocrinology 226.2 (2015): T101-T122.
- Vermeulen, A., S. Ando, and L. Verdonck. “Prolactinomas, testosterone-binding globulin, and androgen metabolism.” The Journal of Clinical Endocrinology & Metabolism 54.2 (1982): 409-412.
- Koehler, Karsten, et al. “Low energy availability in exercising men is associated with reduced leptin and insulin but not with changes in other metabolic hormones.” Journal of sports sciences 34.20 (2016): 1921-1929.
- Cameron, Judy L., et al. “Slowing of pulsatile luteinizing hormone secretion in men after forty-eight hours of fasting.” The Journal of Clinical Endocrinology & Metabolism 73.1 (1991): 35-41.
- Aloi, J. A., et al. “Pulsatile intravenous gonadotropin-releasing hormone administration averts fasting-induced hypogonadotropism and hypoandrogenemia in healthy, normal weight men.” The Journal of Clinical Endocrinology & Metabolism 82.5 (1997): 1543-1548.
- Alemany, Joseph A., et al. “Effects of dietary protein content on IGF-I, testosterone, and body composition during 8 days of severe energy deficit and arduous physical activity.” Journal of applied physiology 105.1 (2008): 58-64.
- Nindl, Bradley C., et al. “Physiological consequences of US Army Ranger training.” Medicine & Science in sports & exercise 39.8 (2007): 1380-1387.
- Friedl, Karl E., et al. “Endocrine markers of semistarvation in healthy lean men in a multistressor environment.” Journal of Applied Physiology (2000).
- Breen, Kellie M., and Pamela L. Mellon. “Influence of stress-induced intermediates on gonadotropin gene expression in gonadotrope cells.” Molecular and cellular endocrinology 385.1-2 (2014): 71-77.
- Luton,Jean-Pierre, et al. “Reversible gonadotropin Deficiency in Male Cushing’s Dusease.” The Journal of Clinical Endocrinology & Metabolism 45.3 (1977): 488-495.
- MacAdams, Michael R., Richard H. White, and Bradley E. Chipps. “Reduction of serum testosterone levels during chronic glucocorticoid therapy.” Annals of internal medicine 104.5 (1986): 648-651.
Main article image by Gerd Altmann from Pixabay.
About the author
Peter Bond is a scientific author with publications on anabolic steroids, the regulation of an important molecular pathway of muscle growth (mTORC1), and the dietary supplement phosphatidic acid. He is the author of several books in Dutch and English, including Book on Steroids and Bond's Dietary Supplements.
3 replies
Loading new replies...
Join the full discussion at the MESO-Rx →